Biosensors
On the biological membranes that cover the cells constituting our bodies, there exist membrane proteins called ion channels (membrane transporters), which play a crucial role in transporting ions essential for maintaining cellular activity. When these ion channels malfunction, it can lead to serious diseases. Therefore, using artificial ion channels to replace the function of the malfunctioning ion channels is expected to lead to the establishment of new therapeutic methods. Artificial ion channels can also be expected to be applied as biosensors that sensitively detect the activity of nerves and muscles.
Artificial Ion Channels
Using DNA origami technology (DNA nanotechnology), we aim to construct artificial ion channels with selective ion permeation functions. With artificial channels made of DNA, the channel structure can be freely designed by designing the DNA base sequence, offering high flexibility in the fabrication method. This allows for flexible design and production based on theoretical design guidelines. In collaboration with experimental groups, we are designing artificial channels from both experimental and theoretical perspectives. Using molecular simulations, we adjust the pore size within the DNA channels, control the selectivity of permeating molecules with modifying molecules, and evaluate the binding stability to lipid bilayers, thereby optimizing theoretical design guidelines.
Six-helix bundle (6HB) DNA nanopore
Research Topic Examples
Influence of Pore Size on Ion Transport in Artificial DNA Channels
Using artificial DNA channels created through DNA nanotechnology, we focus on the transport dependency on pore size in ion transport. We aim to create more functional synthetic channels.
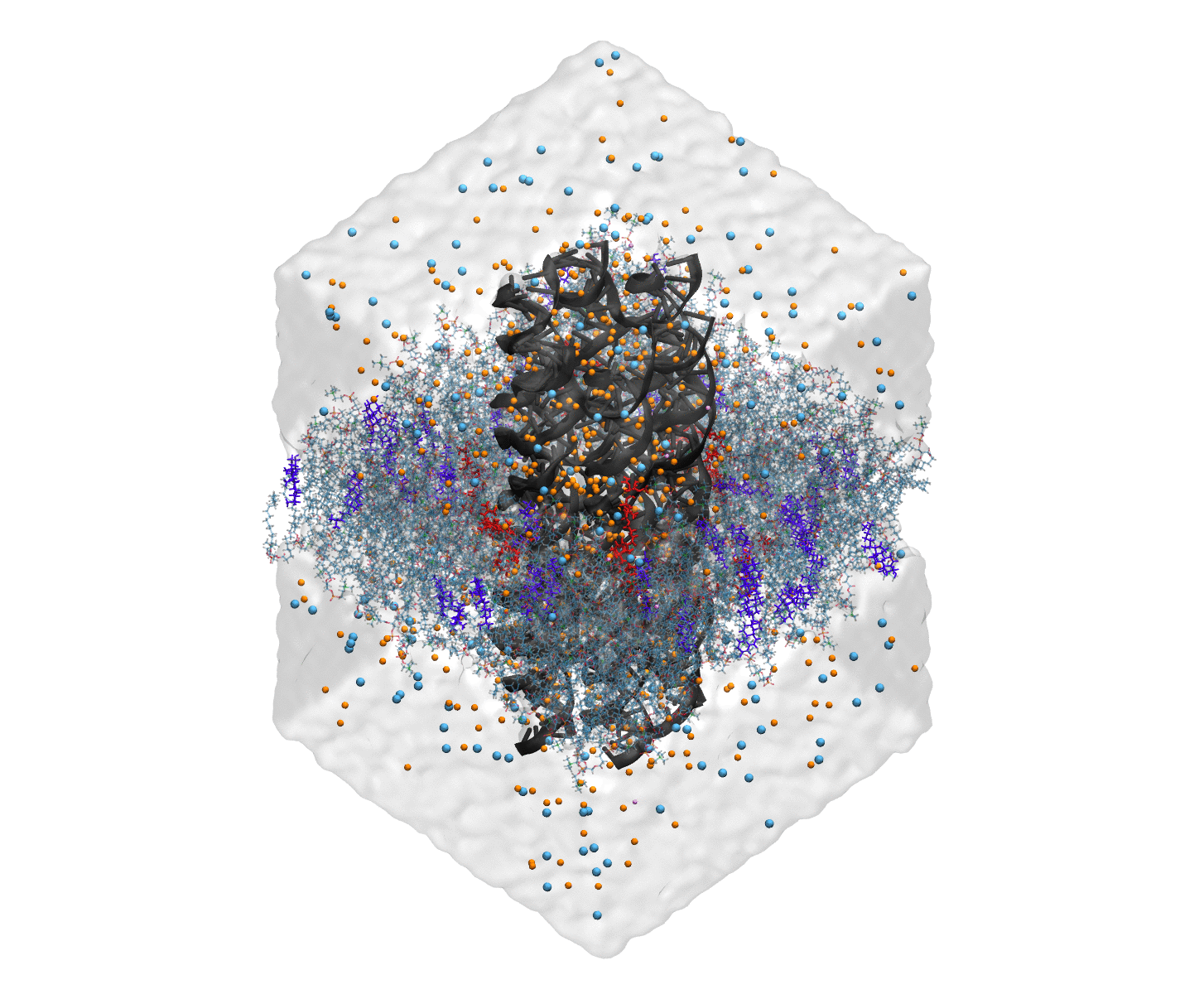
Influence of Functional Groups on Ion Transport in Artificial DNA Channels
By modifying the interior of artificial DNA channels with arbitrary functional groups, we investigate the impact of these groups on ion transport properties. This study focuses on the molecular-level structural and chemical properties within the channel to achieve selective permeability in artificial DNA channels.
Towards the Development of Channels for Selective Proton Transport
The transport of protons (H+) requires consideration of a complex mechanism involving chemical reactions with water molecules, known as the Grotthuss mechanism. In this research, using quantum chemical calculations and a bespoke reactive MD model, we clarify the correlation between the hydrogen bonding network of water molecules inside nanopores and the ion conduction mechanism.
Elucidating the Ion Transport Mechanism of F-/H+ Exchange Transporters (CLCF)
Understanding the structure and function of natural ion channels is crucial in the design of artificial ion channels. Therefore, we aim to elucidate ion transport mechanisms using molecular simulations, targeting various natural ion channels. One such study involves the F-/H+ Exchange Transporters (CLCF), which is crucial for survival functions such as energy production in bacteria. CLCF regulates intracellular fluoride ion concentrations, and significant concentration changes due to channel abnormalities can lead to bacterial death.
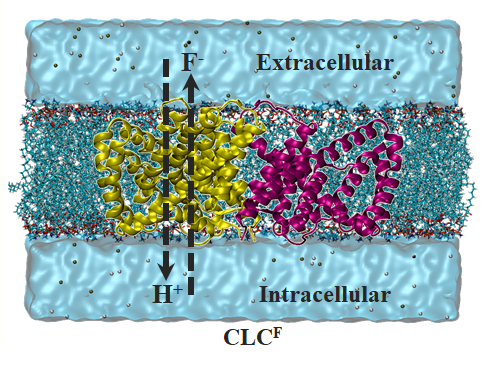
Membrane Insertion
Even if highly functional artificial DNA channels are designed, they cannot exhibit their function without penetrating the membrane. In the latest research, the proportion of experimental artificial DNA molecules that penetrate membranes remains around 20%, and improving yield is also a major challenge. We systematically evaluate the correlation between the interaction characteristics of the DNA channels that drive membrane penetration and the lipid bilayer, including the effects of curvature and electric field due to liposome formation, ion types, and concentrations. We are optimizing the membrane penetration process.
Drug Design
Liquid-Liquid Phase Separation (LLPS)
It is known that proteins and RNA self-assemble to undergo liquid-liquid phase separation, forming droplets or gel-like structures within the cell. Cells are thought to regulate various life phenomena, such as transcription, translation, and signal transduction, by converting biomolecules into droplets. This research is a collaborative study with experimental groups, where we theoretically design artificial molecules based on phase-separating proteins such as elastin-like polypeptides (ELPs). We aim to advance a bottom-up understanding of intracellular phase separation and develop methods to control the function of targeted proteins within the cell by trapping specific molecules in droplets.
ELP coacervate formation
Research Topic Examples
Impact of Amino Acid Residue Incorporation on Phase Separation in Elastin-like Polypeptides (ELPs)
We are conducting theoretical design of molecules based on ELPs that incorporate various amino acid residues. We analyze ion distribution within droplets and intermolecular interactions, aiming to elucidate the mechanism of liquid-liquid phase separation (LLPS) formation under various environmental conditions and to control the uptake of target molecules.
RNA partitioning in the ELP coacervate
Artificial Enzymes
Artificial metalloenzymes, obtained by introducing metal complexes into the internal spaces of proteins, can enhance reaction rates and impart reaction selectivity, adding the characteristics derived from proteins to the catalytic abilities of metal complexes. If synthetic catalysts can be freely integrated into biochemical reaction networks, these catalysts can be promising candidates for new pharmaceuticals. It is crucial to achieve biocompatibility of metal complexes while establishing a more versatile intracellular catalytic reaction system. In this research, as a collaborative study with experimental groups, we aim to support the design of artificial metalloenzymes being developed by the experimental groups from a theoretical perspective using molecular simulations, and strive for more efficient design by merging theoretical and experimental approaches.
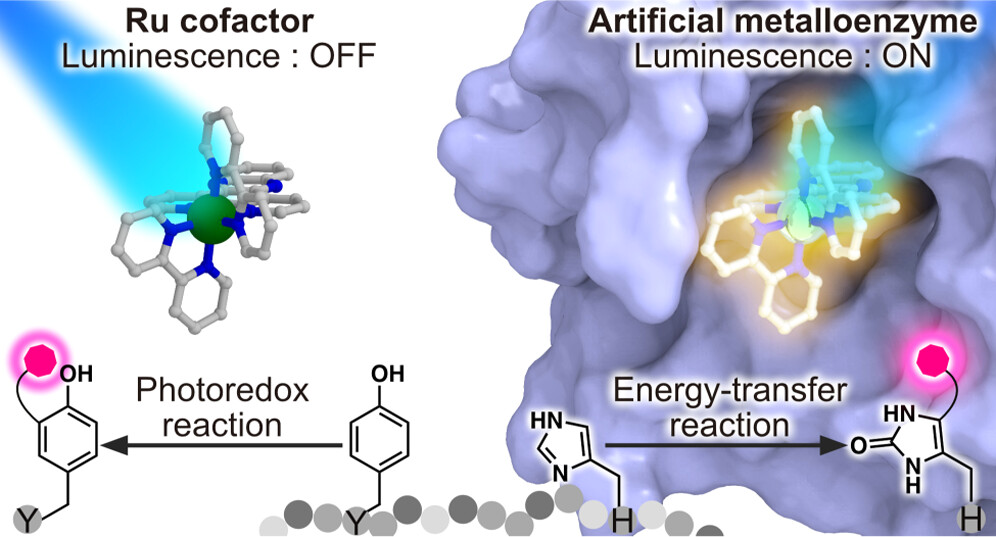
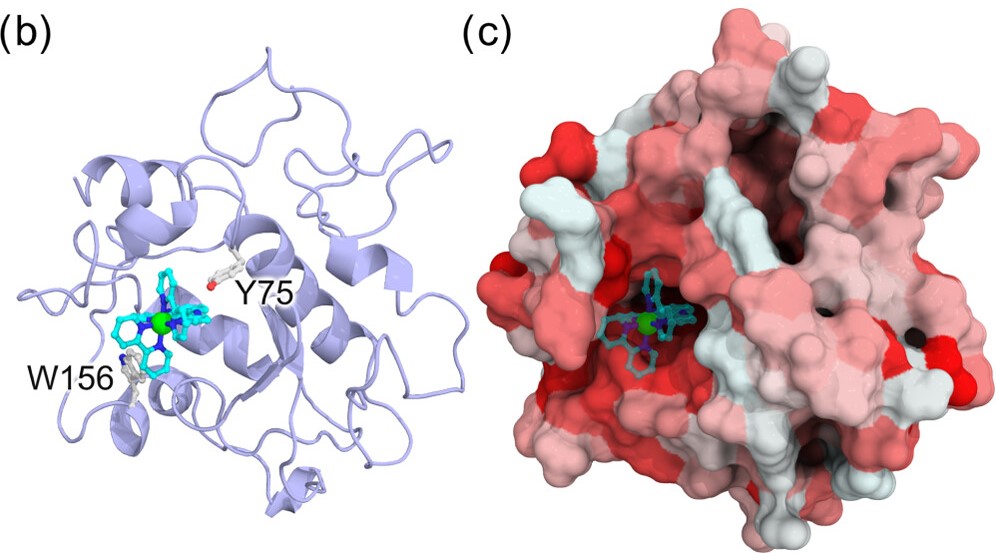
